SOLID STATE IONICS AND BATTERIES
Solid-state lithium (Li) batteries provide possible solutions to the primary problems encountered in traditional Li-ion batteries with liquid electrolytes such as safety and environmental stability. To enable the development of solid-state batteries, we are investigating solid-state electrolytes (SSEs), in particular garnet-type SSEs, which have a high ionic conductivity, excellent environmental stability, and wide electrochemical stability window.
The major challenge for high-performance solid-state batteries is the high solid–solid interfacial impedance between the garnet electrolyte and the electrode materials. By coating the interface between the Li metal anode and the garnet electrolyte with an ultra-thin layer of material (such as Al2O3), we were able to effectively address the interfacial impedance issues at room temperature.
3D garnet frameworks can also find applications in high energy density, high power-density batteries, which is something our group has demonstrated, showing the improved cycling stability and suppressed Li dendrite growth that can be achieved.
We are also collaborating with Prof. Gary Rubloff’s group on the development of solid state batteries using ALD solid state electrolytes.
Recent Papers:
-
Han, X.; Gong, Y.; Fu, K.; He, X.; Hitz, G. T.; Dai, J.; Pearse, A.; Liu, B.; Wang, H.; Rubloff, G.*; Mo, Y.*; Thangadurai, V.*; Wachsman, E. D.*; Hu, L.* Negating interfacial impedance in garnet-based solid-state Li metal batteries. Nature Materials 2017, 16, 572.
-
Fu, K.; Gong, Y.; Liu, B.; Zhu, Y.; Xu, S.; Yao, Y.; Luo, W.; Wang, C.; Lacey, S. D.; Dai, J.; Chen, Y.; Mo, Y.*; Wachsman, E.*; Hu, L.* Toward garnet electrolyte–based Li metal batteries: An ultrathin, highly effective, artificial solid-state electrolyte/metallic Li interface. Science Advances 2017, 3, e1601659.
-
Wang, C.; Gong, Y.; Liu, B.; Fu, K.; Yao, Y.; Hitz, E.; Li, Y.; Dai, J.; Xu, S.; Luo, W.; Wachsman, E. D.*; Hu, L.* Conformal, Nanoscale ZnO Surface Modification of Garnet-Based Solid-State Electrolyte for Lithium Metal Anodes. Nano Letters 2017, 17, 565.

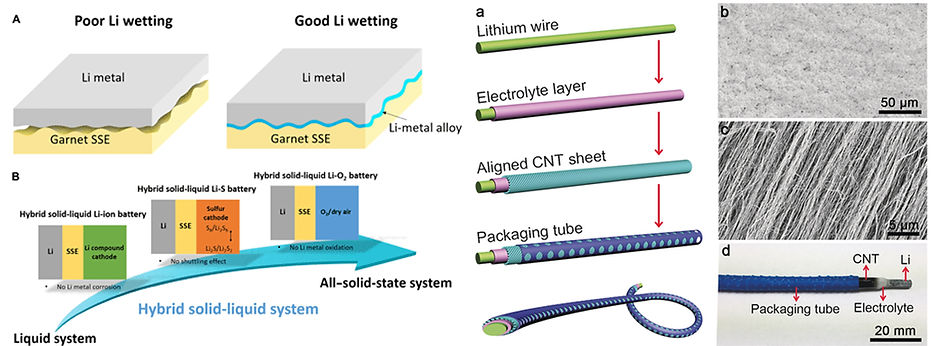
METAL-AIR BATTERIES
The lithium–air battery has been proposed as the next‐generation energy‐storage device with a much higher energy density compared with the conventional lithium‐ion battery. However, lithium–air batteries currently suffer enormous problems including parasitic reactions, low recyclability in air, degradation, and leakage of liquid electrolyte. Besides, they are designed into a rigid bulk structure that cannot meet the flexible requirement in the modern electronics.
Recent Papers:
-
Zhang, Y.; Luo, W.; Wang, C.; Li, Y.; Chen, C.; Song, J.; Dai, J.; Hitz, E. M.; Xu, S.; Yang, C.; Wang, Y.; Hu, L.* High-Capacity, Low-Tortuosity, and Channel-Guided Lithium Metal Anode. Proceedings of the National Academy of Sciences 2017, 114 (14), 3584–3589.
-
Fu, K.K.; Gong, Y.; Hitz, G.T.; McOwen, D.W.; Li, Y.; Xu, S.; Wen, Y.; Zhang, L.; Wang, C.; Pastel, G.; Dai, J.; Hu, L.* Three-dimensional bilayer garnet solid electrolyte based high energy density lithium metal–sulfur batteries. Energy & Environmental Science 2017, 10(7), pp.1568-1575.
-
Wang, C.; Gong, Y.; Liu, B.; Fu, K.; Yao, Y.; Hitz, E.; Li, Y.; Dai, J.; Xu, S.; Luo, W.; Wachsman, E.D.; Hu, L.* Conformal, nanoscale ZnO surface modification of garnet-based solid-state electrolyte for lithium metal anodes. Nano letters 2017, 17(1), pp.565-571.
-
Xu, S.; Yao, Y.; Guo, Y.; Zeng, X.; Lacey, S.D.; Song, H.; Chen, C.; Li, Y.; Dai, J.; Wang, Y.; Chen, Y.; Hu, L.* Textile inspired lithium–oxygen battery cathode with decoupled oxygen and electrolyte pathways. Advanced Materials 2018, 30(4), p.1704907.
LARGE SCALE ENERGY STORAGE
Revolutionary new high energy density batteries for electric vehicle, consumer electronics, and grid-scale energy storage require low-cost, long cycling, and scalable manufacturing. In particular, grid-scale energy storage is an essential component for smart grids to integrate renewable energy sources (solar, wind) for a self-sustainable community.
Our group is interested in energy storage technologies that use inexpensive materials with the potential to meet target cost values, including sodium ion batteries (NIBs), wood-based aqueous devices, and flow batteries. We pursue basic scientific studies to understand the electrochemical performance-structure relationship and engineering efforts to achieve high performance towards practical applications in this area. Examples of the former include cellulose nanofibers as electrolyte reservoirs and stress-releasing matrices for NIBs. Examples of the latter include wood-based NIBs and supercapacitors where nature-inspired design was utilized to construct low-tortuosity and highly loaded ultrathick energy storage devices.
We invented paper-based sodium ion batteries that can be potentially manufactured using existing paper manufacturing infrastructure. We are working with cellulose companies to further push the technology for practical applications.
Recent Papers:
-
Chen, C.; Zhang, Y.; Li, Y.; Dai, J.; Song, J.; Yao, Y.; Gong, Y.; Kierzewski, I.; Xie, J.; Hu, L.* All-wood, low tortuosity, aqueous, biodegradable supercapacitors with ultra-high capacitance. Energy & Environmental Science 2017, 10, 538-545.
-
Shen, F.; Luo, W.; Dai, J.; Yao, Y.; Zhu, M.; Hitz, E.; Tang, Y.; Chen, Y.; Sprenkle, V. L.; Li, X.; Hu, L.* Ultra‐Thick, Low‐Tortuosity, and Mesoporous Wood Carbon Anode for High‐Performance Sodium‐Ion Batteries. Advanced Energy Materials 2016, 6.
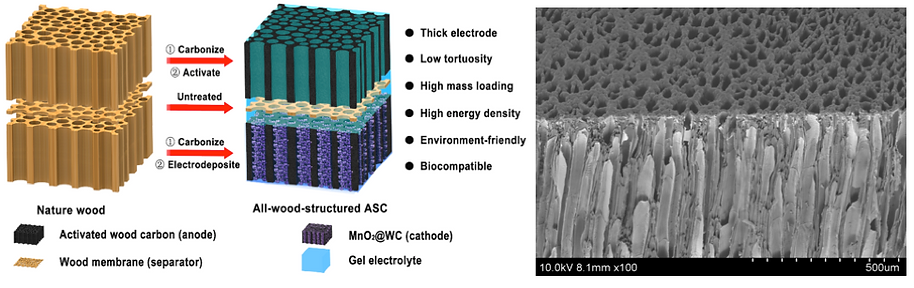
BATTERIES WITH UNCONVENTIONAL FORMATS
With the rapid development of flexible electronics, batteries with high performance as well as superior flexibility and durability for integration into flexible electronics have become increasingly more desirable. Our group is interested in using low-dimensional materials, such as carbon nanotubes and graphene, combined with flexible structural materials such as papers and textiles to develop flexible batteries.
For wearable applications, fiber-based batteries have attracted more attention due to their potential integration into wearable electronics, as they can be potentially woven into a garment without compromising its thermal comfort and aesthetic appearance. Recently, we developed printable inks to make an entire battery using 3D-printed flexible and stretchable fibers, which exhibited good electrochemical performance and good wearable capabilities.
Our group is also working on solid state flexible batteries for internet of things (IoT) applications.
Recent Papers:
-
Fu, K.; Gong, Y.; Dai, J.; Gong, A.; Han, X.; Yao, Y.; Wang, C.; Wang, Y.; Chen, Y.; Yan, C.; Li, Y.; Wachsman, E.; Hu, L.* Flexible, solid-state, ion-conducting membrane with 3D garnet nanofiber network for lithium batteries, Proceedings of the National Academy of Sciences, 2016, 113, 7094.
-
Wang, Y.; Chen, C.; Xie, H.; Gao, T.; Yao, Y.; Pastel, G.; Han, X.; Li, Y.; Zhao, J.; Fu, K.; Hu, L.* 3D-Printed All-Fiber Li-Ion Battery toward Wearable Energy Storage, Advanced Functional Materials, 2017, 27, 1703140
-
Fu, K.; Cheng, J.; Li, T.; Hu, L.* Flexible Batteries: From Mechanics to Devices, ACS Energy Letters, 2016, 1, 1065
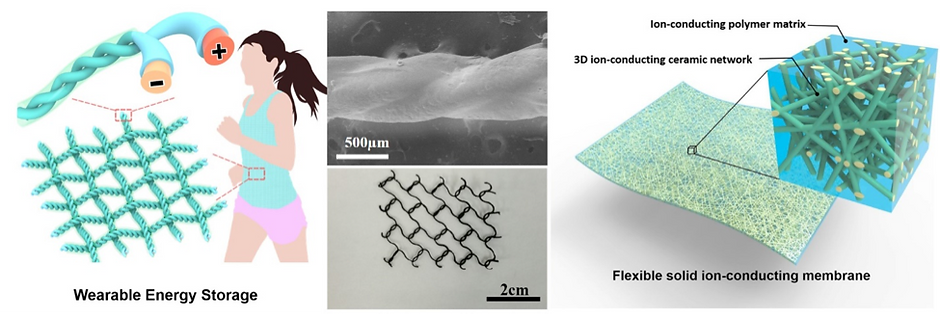
TRANSIENT BATTERY
Transient electronics requires materials, devices, and systems to be capable of disappearing with minimal or non-traceable remains over a period of stable operation. Electronics with the capability of disintegrating or vanishing after stable operation are an interesting research topic and have attracted increasing attention. In recent years, transience technology has been extended to intelligence applications, bioelectronics, environmental monitoring systems, and energy harvesting and storage. As an important part of transient electronics, we have investigated the fundamental properties of degradable materials and demonstrated a transient rechargeable power supply with high power, high capacity, and fast transience capabilities.
Recent Papers:
-
Fu, K.; Wang, Z.; Dai, J.; Carter, M.; Hu, L. * Transient Electronics: Materials and Devices, 2016, Chemistry of Materials (Invited), 28, 3527.
-
Wang, Z.’ Fu, K.; Liu, Z.; Yao, Y.; Dai, J.; Wang, Y.; Liu, B.; Hu, L. * Design of High Capacity Dissoluble Electrodes for All Transient Batteries, Advanced Functional Materials, 2017, 27, 1605724.
-
Fu, K.; Wang, Z.; Yao, C.; Liu, Z.; Yao, Y.; Dai, J.; Hitz, E.; Yang, Y.; Luo, W.; Chen, Y.; Kim, M.; Hu, L. * All-component Transient Lithium-Ion Batteries, Advanced Energy Materials, 2016, 6, 1502496.
-
Fu, K.; Liu, Z.; Yao, Y.; Wang, Z.; Zhao, B.; Luo, W.; Dai, J.; Lacey, S.; Zhou, L.; Shen, F.; Kim, M.; Swafford, L.; Sengupta, L.; Hu, L. * Transient Rechargeable Batteries Triggered by Cascade Reactions, Nano. Letter, 2015, 15, 4664

NANO-IONIC AND NANO-BATTERY
Our group developed a novel method for investigating the mechanical properties of nanoparticles for energy storage applications by in situ TEM and demonstrated that an ALD-Al2O3 coating offers a dynamic protection to maintain the structural integrity of these nanoparticles during the charge/discharge process of a cell.
We have also designed a planar nano-battery and measured in situ optical transmittance spectra and electrical transport properties of ultrathin graphite and MoS2 flakes simultaneously during electrochemical lithiation/delithiation. We achieved both high transparency and conductivity in lithiated devices, which is useful for transparent conductors. We further investigated the solid electrolyte interphase (SEI) formation at the nanoscale and measured the mechanical properties of the SEI layer by in situ AFM. Understanding ion insertion/extraction in battery materials at the nanoscale under controlled electrical and/or chemical potentials is critical for improving utilization of these materials in practical devices.
Currently, we are interested in the ionic, electronic and mechanical properties of a battery system at the nanoscale. We are also exploring planar nano-batteries that enable various in situ investigation of nanoscale ion intercalation (nano-ionic) to independently diagnose the failure mechanisms of all-solid-state batteries.
Recent Papers:
-
Han, X.; Liu, Y.; Jia, Z.; Chen, Y.-C.; Wan, J.; Weadock, N.; Gaskell, K. J.; Li, T.; Hu, L.,* Atomic-Layer-Deposition Oxide Nanoglue for Sodium Ion Batteries. Nano Lett. 2014, 14 (1), 139-147;
-
Weadock, N.; Varongchayakul, N.; Wan, J.; Lee, S.; Seog, J.; Hu, L.* Determination of mechanical properties of the SEI in sodium ion batteries via colloidal probe microscopy. Nano Energy 2013, 2 (5), 713-719;
-
Wan, J.; Bao, W.; Liu, Y.; Dai, J.; Shen, F.; Zhou, L.; Cai, X.; Urban, D.; Li, Y.; Jungjohann, K.; Fuhrer, M. S.; Hu, L.* In Situ Investigations of Li-MoS2 with Planar Batteries. Adv. Energy Mater. 2015, 5 (5), 1401742;
-
Bao, W.; Wan, J.; Han, X.; Cai, X.; Zhu, H.; Kim, D.; Ma, D.; Xu, Y.; Munday, J. N.; Drew, H. D.; Fuhrer, M. S.; Hu, L.* Approaching the limits of transparency and conductivity in graphitic materials through lithium intercalation. Nat. Commun. 2014, 5, 4224;
-
Lacey, S. D.; Wan, J.; Cresce, A. v. W.; Russell, S. M.; Dai, J.; Bao, W.; Xu, K.; Hu, L.* Atomic Force Microscopy Studies on Molybdenum Disulfide Flakes as Sodium-Ion Anodes. Nano Lett. 2015, 15 (2), 1018-1024.

IN SITU NEUTRON DEPTH PROFILING FOR SOLID STATE BATTERIES
We use neutron depth profiling (NDP), a nondestructive and uniquely Li-sensitive technique, to reveal the interfacial behavior of garnet SSE in contact with metallic Li through in situ monitoring of Li plating-stripping processes. The NDP measurement demonstrates predictive capabilities for diagnosing short-circuits in garnet-based solid state batteries. We are interested in developing stable SSEs and SSE-electrode interfaces for high energy and power density solid state batteries with the help of the in situ NDP diagnosis technique.
Recent Papers:
-
Wang, C.; Gong, Y.; Dai, J.; Zhang, L.; Xie, H.; Pastel, G.; Liu, B.; Wachsman, E.*; Wang, H.*; Hu, L.* In Situ Neutron Depth Profiling of Lithium Metal–Garnet Interfaces for Solid State Batteries. Journal of the American Chemical Society, 139(40), pp.14257-14264.
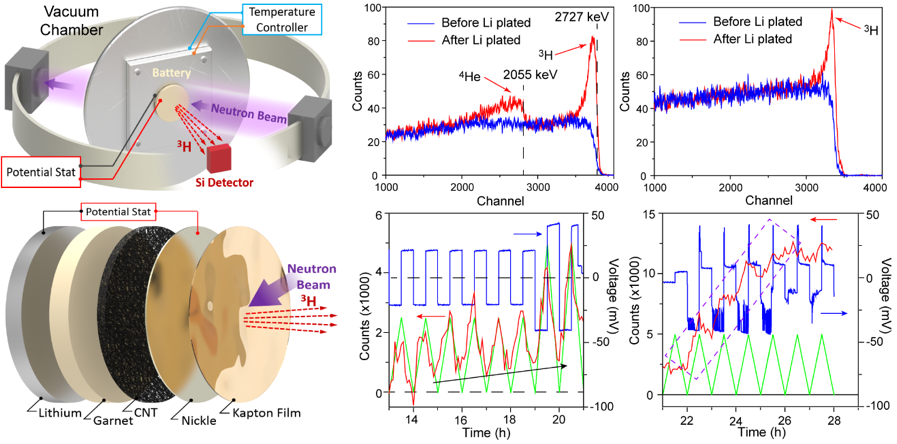